Professor Franklin Dollar reflects on the 2023 Nobel Prize in Physics
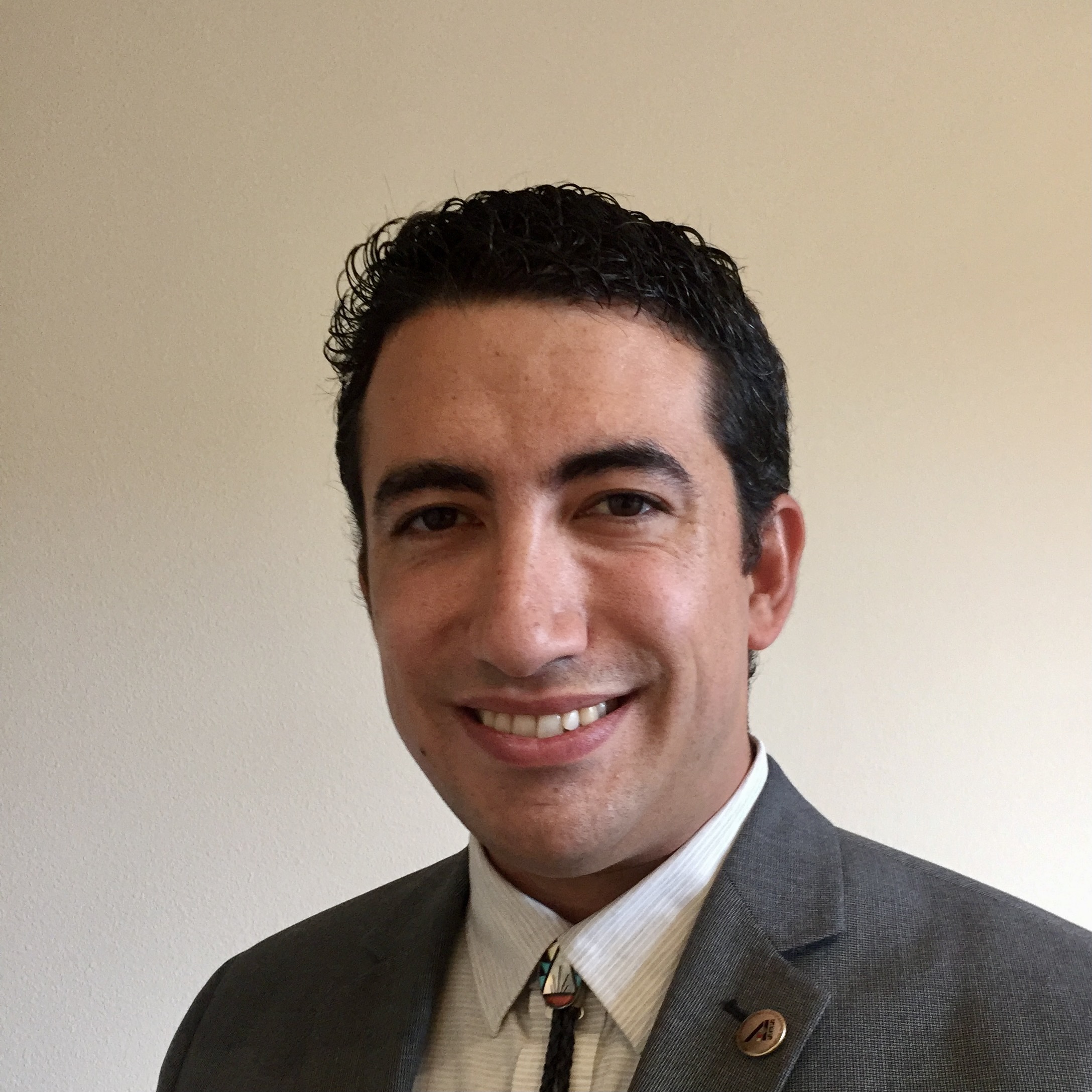
Dollar works to further research into high-intensity laser physics, as well as to make his field more inclusive, diverse and equitable.
The 2023 Nobel Prize in Physics went to three physicists: Anne L’Huiller of Lund University in Sweden, Pierre Augustini of the Ohio State University and Ferenc Krausz of the Max Planck Institute of Quantum Optics and Ludwig Maximilian University of Munich. The prize honors work each has done to make the field of attosecond science a reality — a field that deals with the tools and techniques it takes to generate pulses of light that are mere billionths of a second in length. Such light pulses are so brief that researchers can use them to take pictures of events that take place over ultra-short timescales, including the movements of electrons around atoms.
Many scientists in the UC Irvine Department of Physics & Astronomy and Department of Chemistry do attosecond science research, including Professors Shaul Mukamel, Ara Apkarian, Toshi Tajima and Franklin Dollar.
Dollar and his lab do work to develop attosecond-scale light sources. UCI Physical Sciences Communications caught up with Dollar to find out more about the significance of this year’s Nobel Prize in Physics, and how his own research relates to the work it recognizes.
Dollar is the Associate Dean of Graduate Studies at the UCI School of Physical Sciences, and the UCI site lead of a National Science Foundation Science & Technology Center called STROBE, which is developing new ways of imaging using attosecond light pulses.
LVWJ: As an attosecond science expert, can you explain why you think the work of these scientists is worthy of a Nobel Prize?
FD: The field of attosecond science was a direct result of the seminal works by these scientists. When we take a picture of something, how clear the picture is depends on how fast the camera flash is. Try to take a picture of a sports event without a flash and the players are all blurry. The faster the flash, the faster dynamics you can capture with your camera. With the development of attosecond light, which is light that flashes for a billionth of a billionth of a second, you can now see the fastest events in science. This is the natural timescale of the electron state around an atom. If you want to directly observe atomic and molecular processes, such as chemistry or magnetism, you need light that is this fast.
LVWJ: How does the work relate to your own research? Are you trying to achieve similar things, or are your motivations slightly different?
FD: This Nobel work is some of the most foundational work done in the field of attosecond science. My own work is in the experimental development of these types of light sources, both within the regime that the Laureates operate and at higher laser intensities. The goal of my work is to understand the underlying physical processes of how to make such light, as well as how to make the light sources more useful. For instance, we performed an experiment in which we could measure the magnetization state of nanoscale magnetic foils on attosecond timescales. We are also looking into ways of making the light more accessible, both by increasing the efficiency of the generation process and increasing the range of wavelengths that we can make. The basic process of making those light pulses is the same as it was in the Nobel-winning work from decades ago.
LVWJ: How much of a leap forward in your field does the Nobel work represent? Can you put it into context for me?
FD: In my lab, we perform a demo to visitors in which we take our infrared laser beam and focus it in the air. Our eyes cannot see the infrared, so the laser is essentially invisible to us. However, when focused, the intensity is high enough to enable nonlinear optical processes that can change the color of the laser from the infrared to the visible. In other words, the invisible laser is focused to a spot and a rainbow comes out of seemingly nothing. This process is similar to what goes on in the Nobel work. We focus the laser on gas targets in a controlled manner that enables us to shift the frequencies to much higher energies — energies required to sustain an attosecond pulse.
At the intensities we are used to, even those made by focusing the sun with a magnifying glass, electrons stay stuck to their parent atoms. We discovered pretty quickly that if a laser is intense enough, we can pull apart matter in a process called ionization, where the electron is pulled away from the ion. The Nobel work examined what is possible when you are just below such an intensity threshold. The electron is “pulled” as far away from the atom as possible without being ionized. When the electron is “let go,” it “snaps” back into place and makes a high frequency photon. While the process is quantum mechanical in nature, it is not dissimilar from a guitar string pulled to the breaking point.
LVWJ: Anything else about the importance of the award you can put into context?
FD: Five years ago we had the Nobel Prize in Physics awarded for high intensity lasers, and now we have another that wouldn't be possible without ultrafast lasers. I think it really highlights how much can happen in a short period of time with new and rapidly evolving laser technologies.