Why the Big Bang Produced Something Rather Than Nothing
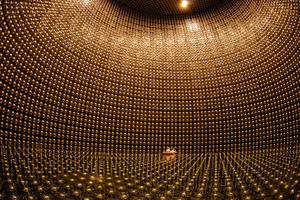
The Super-Kamiokande Neutrino Observatory, located more than 3,000 feet below Mount Ikeno near the city of Hida, Japan.
Scientists on Wednesday announced that they were perhaps one step closer to understanding why the universe contains something rather than nothing.
Part of the blame, or the glory, they say, may belong to the flimsiest, quirkiest and most elusive elements of nature: neutrinos. These ghostly subatomic particles stream from the Big Bang, the sun, exploding stars and other cosmic catastrophes, flooding the universe and slipping through walls and our bodies by the billions every second, like moonlight through a screen door.
Neutrinos are nature’s escape artists. Did they help us slip out of the Big Bang? Perhaps. Recent experiments in Japan have discovered a telltale anomaly in the behavior of neutrinos, and the results suggest that, amid the throes of creation and annihilation in the first moments of the universe, these particles could have tipped the balance between matter and its evil-twin opposite, antimatter.
As a result, a universe that started out with a clean balance sheet — equal amounts of matter and antimatter — wound up with an excess of matter: stars, black holes, oceans and us.
An international team of 500 physicists from 12 countries, known as the T2K Collaboration and led by Atsuko K. Ichikawa of Kyoto University, reported in Nature that they had measured a slight but telling difference between neutrinos and their opposites, antineutrinos. Although the data is not yet convincing enough to constitute solid proof, physicists and cosmologists are encouraged that the T2K researchers are on the right track.
“This is the first time we got an indication of the CP violation in neutrinos, never done before,” said Federico Sánchez, a physicist at the University of Geneva and a spokesman for the T2K collaboration, referring to the technical name for the discrepancy between neutrinos and antineutrinos. “Already this is a real landmark.”
But Dr. Sánchez and others involved cautioned that it is too early to break out the champagne. He pointed out that a discrepancy like this was only one of several conditions that Andrei Sakharov, the Russian physicist and dissident winner of the Nobel Peace Prize in 1975, put forward in 1967 as a solution to the problem of the genesis of matter and its subsequent survival.
Not all the conditions have been met yet. “This is just one of the ingredients,” Dr. Sánchez said. Nobody knows how much of a discrepancy is needed to solve the matter-antimatter problem. “But clearly this goes in the right direction,” he said.
In a commentary in Nature, Silvia Pascoli of Durham University in England and Jessica Turner of the Fermi National Accelerator Laboratory in Batavia, Ill., called the measurement “undeniably exciting.”
“These results could be the first indications of the origin of the matter-antimatter asymmetry in our universe,” they wrote.
The Japan team estimated the statistical significance of their result as “3-sigma,” meaning that it had one chance in 1,000 of being a fluke. Those odds may sound good, but the standard in physics is 5-sigma, which would mean less than a one-in-a-million chance of being wrong.
“If this is correct, then neutrinos are central to our existence,” said Michael Turner, a cosmologist now working for the Kavli Foundation and not part of the experiment. But, he added, “this is not the big discovery.”
Joseph Lykken, deputy director for research at Fermilab, said he was cheered to see a major science result coming out during such an otherwise terrible time. “The T2K collaboration has worked really hard and done a great job of getting the most out of their experiment,” he said. “One of the biggest challenges of modern physics is to determine whether neutrinos are the reason that matter got an edge over antimatter in the early universe.”
We are the beauty mark of the universe
The Russian physicist Andreï Sakharov at home in Moscow in 1974.Credit...Christian Hirou/Gamma-Rapho, via Getty Images
In a perfect universe, we would not exist.
According to the dictates of Einsteinian relativity and the baffling laws of quantum theory, equal numbers of particles and their opposites, antiparticles, should have been created in the Big Bang that set the cosmos in motion. But when matter and antimatter meet, they annihilate each other, producing pure energy. (The concept, among others, is what powers the engines of the Starship Enterprise.) Therefore, the universe should be empty of matter.
That didn’t happen, quite. Of the original population of protons and electrons in the universe, roughly only one particle in a billion survived the first few seconds of creation. That was enough to populate the skies with stars, planets and us.
In 1967 Dr. Sakharov laid out a prescription for how matter and antimatter could have survived their mutual destruction pact. One condition is that the laws of nature might not be as symmetrical as physicists like Einstein assumed.
In a purely symmetrical universe, physics should work the same if all the particles changed their electrical charges from positive to negative or vice versa — and, likewise, if the coordinates of everything were swapped from left to right, as if in a mirror. Violating these conditions — called charge and parity invariance, C and P for short — would cause matter and antimatter to act differently.
In 1957, Tsung-Dao Lee of Columbia University and Chen Ning Yang, then at Institute for Advanced Study, won the Nobel Prize in Physics for proposing something along these lines. They suggested that certain “weak interactions” might violate the parity rule, and experiments by Chien-Shiung Wu of Columbia (she was not awarded the prize) confirmed the theory. Nature, in some sense, is left-handed.
In 1964, a group led by James Cronin and Val Fitch, working at the Brookhaven National Laboratory on Long Island, discovered that some particles called kaons violated both the charge and parity conditions, revealing a telltale difference between matter and antimatter. These scientists also won a Nobel.
Hints of a discrepancy between matter and antimatter have since been found in the behavior of other particles called B mesons, in experiments at CERN and elsewhere.
“In the larger picture, CP violation is a big deal,” Dr. Turner said. “It is why we are here!”
Both kaons and B mesons are made of quarks, the same kinds of particles that make up protons and neutrons, the building blocks of ordinary matter. But so far there is not enough of a violation on the part of quarks, by a factor of a billion, to account for the existence of the universe today.
Neutrinos could change that. “Many theorists believe that finding CP violation and studying its properties in the neutrino sector could be important for understanding one of the great cosmological mysteries,” said Guy Wilkinson, a physicist at Oxford who works on CERN’s LHCb experiment, which is devoted to the antimatter problem. Chief among those mysteries, he said: “Why didn’t all matter and antimatter annihilate in the Big Bang?”
Help from the ghost side
A bubble chamber showing muon neutrino traces, taken Jan. 16, 1978, at the Fermi National Accelerator Laboratory outside Chicago.Credit...Fermilab/Science Source
Neutrinos would seem to be the flimsiest excuse on which to base our existence — “the most tiny quantity of reality ever imagined by a human being,” a phrase ascribed to Frederick Reines, of the University of California, Irvine, who discovered neutrinos.
They entered the world stage in 1930, when the theorist Wolfgang Pauli postulated their existence to explain the small amount of energy that goes missing when radioactive decays spit out an electron. Enrico Fermi, the Italian physicist, gave them their name, “little neutral one,” referring to their lack of an electrical charge. In 1955 Dr. Reines discovered them emanating from a nuclear reactor.; he eventually won a Nobel Prize.
Second to photons, which compose electromagnetic radiation, neutrinos are the most plentiful subatomic particles in the universe, famed for their ability to waft through ordinary matter like ghosts through a wall. They are so light that they have yet to be reliably weighed.
But that is just the beginning of their ephemeral magic. In 1936, physicists discovered a heavier version of the electron, called a muon; this shattered their assumption that they knew all the elementary particles. “Who ordered that?” the theorist I.I. Rabi quipped. Further complicating the cosmic bookkeeping, the muon also came with its own associated neutrino, called the muon neutrino, discovered in 1962. That led to another Nobel.
Another even heavier variation on the electron, called the tau, was discovered by Martin Perl and his collaborators in experiments at the Stanford Linear Accelerator Center in the 1970s. Dr. Perl shared the Nobel in 1995 with Dr. Reines.
Physicists have since learned that every neutrino is a blend of three versions, each of which is paired with a different type of electron: the ordinary electron that powers our lights and devices; the muon, which is fatter; and, the tau, which is fatter still. Nobody really knows how these all fit together.
Adding to the mystery, as neutrinos travel about on their ineffable trajectories, they oscillate between their different forms “like a cat turning into a dog,” Dr. Reines once said. That finding was also rewarded with a Nobel. An electron neutrino that sets out on a journey, perhaps from the center of the sun, can turn into a muon neutrino or a tau neutrino by the time it hits Earth.
By the laws of symmetry, antineutrinos should behave the same way. But do they? Apparently not quite. And on that question may hang a tale of cosmic proportions.
Test-driving neutrinos
A mock-up of the more than 13,000 photomultiplier tubes inside the Super-Kamiokande neutrino detector.Credit...Enrico Sacchetti/Science Source
The T2K experiment, which stands for Tokai to Kamioka, is designed to take advantage of these neutrino oscillations as it looks for a discrepancy between matter and antimatter. Or in this case, between muon neutrinos and muon antineutrinos.
Since 2014, beams of both particles have been generated at the J-PARC laboratory in Tokai, on the east coast of Japan, and sent 180 miles through the earth to Kamioka, in the mountains of western Japan.
There they are caught (some of them, anyway) by the Super-Kamiokande neutrino detector, a giant underground tank containing 50,000 tons of very pure water. The tank is lined with 13,000 photomultiplier tubes, which detect brief flashes of light when neutrinos speed through the tank.
A predecessor to this tank made history on Feb. 23, 1987, when it detected 11 neutrinos streaming from a supernova explosion in the Large Magellanic Cloud, a nearby galaxy.
The scientists running the T2K experiment alternate between sending muon neutrinos and muon antineutrinos — measuring them as they depart Tokai and then measuring them again on arrival in Kamioka, to see how many have changed into regular old electron neutrinos. If nature and neutrinos are playing by the same old-fashioned symmetrical rules, the same amount of change should appear in both beams.
On Wednesday, in the abstract to a rather statistically dense paper, the authors concluded: “Our results indicate CP violation in leptons and our method enables sensitive searches for matter-antimatter asymmetry in neutrino oscillations using accelerator-produced neutrino beams.”
Asked to summarize the result, Dr. Sánchez, a team spokesman, said, “In relative terms more neutrino muons going to neutrino electrons than antineutrino muons going to antineutrino electrons.”
n other words, matter was winning. This was a step in the right direction but, Dr. Sánchez cautioned, not enough to guarantee victory in the struggle to understand our existence. The big thing, he said, is that the experiment has definitely shown that the neutrinos violate the CP symmetry. Whether they violate it enough is not yet known.
“For a long time theorists have been discussing if CP violation in neutrinos would be enough,” Dr. Sánchez said. “The general agreement now is that it does not seem to be sufficient. But this is just modeling, and we might be wrong.”
The Dune horizonWorkers prepared the Large Hadron Collider at CERN in Switzerland for a shutdown period spanning two years in 2019.Credit...Maximilien Brice and Julien Marius Ordan/CERN, via Science Source
More and larger experiments are in the works. Among them is the Deep Underground Neutrino Experiment, or DUNE, a collaboration between the U.S. and CERN. In it, neutrinos will be beamed 800 miles from Fermilab in Illinois to a giant underground detector at the Sanford Underground Research Facility, located in an old gold mine in Lead, S.D., to study how the neutrinos oscillate.
“The T2K/SuperK result does not remove the need for the future experiments,” Dr. Wilkinson of CERN said. “Rather, it encourages us that we are on the right track and to look forward to the conclusive results that we expect to get from these new projects.”
He added, “What the Nature paper tells us is that existing experiments have more sensitivity than was previously thought.”
Dr. Lykken, the deputy director of Fermilab, said, “Now we have a good hint that the DUNE experiment will be able to make a definitive discovery of CP violation relatively soon after it turns on later in this decade.”
The present situation reminded him of the days a decade ago, when physicists were getting ready to turn on the Large Hadron Collider, CERN’s world-beating $10 billion experiment. There were good hints in the data that the long sought Higgs boson, a quantum ghost of a particle that imbues other particles with mass, might be in reach. “Lo and behold those hints were proven correct at the L.H.C.,” Dr. Lykken said.