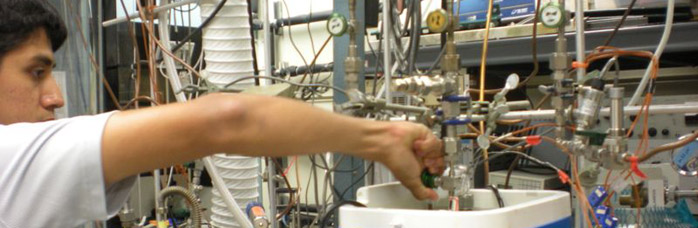
Van Der Waals Complexes
SPECTROSCOPY & DYNAMICS OF VAN DER WAALS COMPLEXES
Noble gas-halogen clusters have been found to be insightful systems for studying many dynamical processes available to larger systems. For example, they are excellent prototypes for studying vibrational predissociation. This process occurs when the initial quasi-bound complex dissociates into free halogen and noble gas atom by the transfer of excess halogen stretching energy to the noble gas-halogen van der Waals bond (Fig). Depending upon the parameters of the noble gas-halogen potential energy surface, the vibrational predissocation can be direct, with the initial bound state coupling directly to the continuum of product states, or intramolecular vibrational energy redistribution (IVR) can mediate the dissociation process, with the excess halogen vibrational energy first going into excited van der Waals modes.
Depending upon the parameters of the noble gas-halogen potential energy surface, the vibrational predissocation can be direct, with the initial bound state coupling directly to the continuum of product states, or intramolecular vibrational energy redistribution (IVR) can mediate the dissociation process, with the excess halogen vibrational energy first going into excited van der Waals modes.
Depending upon the parameters of the noble gas-halogen potential energy surface, the vibrational predissocation can be direct, with the initial bound state coupling directly to the continuum of product states, or intramolecular vibrational energy redistribution (IVR) can mediate the dissociation process, with the excess halogen vibrational energy first going into excited van der Waals modes.
We have made the first spectroscopic observations of the T-shaped ArBr2 complex and characterized its vibrational predissociation dynamics in the B electronic state of Br2 for initial Br2 vibrational levels νi = 14 to 25, using time-dependent pump-probe spectroscopy.
The observed vibrational predissociation dynamics are found to be in the sparse regime of IVR.
Figure: IVR in the sparse regime for ArBr2, with Δν=-3 being the first open product channel. Each potential
energy surface represents the Ar-Br2 interaction for Br2 in a particular vibrational energy level, and the lines
represent van der Waals modes within each manifold of Br2 vibrational levels. Because the binding energy of the
Ar-Br2 van der Waals bond is much larger than the spacing between adjacent Br2 vibrational levels, the initial van der Waals mode in the νi manifold must couple to the lower van der Waals modes of the νi –1 manifold, where the density of states is much less. Therefore, the dynamics become much more sensitive to the details of the initial state, as only slight changes in energy can have drastic changes in the coupling strength.
Experimental Setup:
The independently tunable pump and probe pulses are generated using a dual OPO/OPA system pumped by a single mode-locked picosecond Nd:YAG laser. The output pulses are ~15 ps in duration with a near Fourierlimited frequency resolution of ~ 2 cm-1.
The clusters are formed by passing an Ar/He carrier gas at 40 psig over bromine held at-20 °C, then having this mixture expand into a vacuum chamber held at 10-6 torr through apulsed valve 150 μm in diameter. The pump and probe pulses intersect the free jet expansion, and the resulting fluorescence is recorded by a PMT.
Types of Spectra:
The pump pulse is tuned to prepare the ArBr2 with the desired amount of Br2 vibrational energy in the B electronic state of Br2. The probe pulse comes after a time delay controlled by the delay stage to excite the products of the ArBr2 vibrational predissociation, free Br2 (B, ?i+??), to the Br2 E state. The fluorescence from the E state is the signal recorded by the PMT. Three types of spectra are recorded using this pump-probe technique:
1. Excitation Spectra—In these spectra, the probe pulse is held fixed while the pump laser is scanned. From these the ArBr2 pump transitions are found.
2. Probe Spectra—To identify the products of ArBr2 vibrational predissociation, the pump laser is fixed on the ArBr2 pump transition while the probe laser is scanned.
3. Delay Scans- The formation time of the Br2 products from the ArBr2 are determined by fixing the pump laser on the ArBr2 pump transition, the probe laser on a Br2 product B? E transition, and changing the time delay between the two pulses by moving the delay stage.
*Time and frequency resolved dynamics of ArBr2, Jose A. Cabrera, Craig R. Bieler, Natalie McKinney, Wytze van der Weer, Jordan Pio, Octavio Roncero,& Kenneth C. Janda, Journal of Chemical Physics, 127, 164309 (2007).